Advanced Research WRF
High Resolution
Simulations of the
Inner Core Structure of Hurricanes Katrina, Rita and
Wilma (2005)
The Hurricane Group of the
Mesoscale
and Microscale Meteorology (MMM) division of the National
Center for Atmospheric Research (NCAR) produced
real-time
prediction and retrospective research simulations of
Atlantic and
eastern North Pacific tropical cyclones (TCs) using the
Advanced
Research core of the Weather
Research and
Forecasting model (WRF) from 2004 to 2007,
inclusive.
(See Davis
et al. 2008
for a full description of the model setup and parameters.)
Verification
of the intensity and track errors of the WRF forecasts has
shown that
the 4 km simulations perform as well as, and occasionally
superior to, the National Hurricane
Center (NHC)
official forecasts and other
operational forecast systems.
2005
intensity (kt) and position (n mi) errors for the
Advanced Hurricane
WRF (AHW) forecasts run with an inner moving nest of
4 km (AHW4).
Results from
other
forecast techniques are
defined as
the NHC Official
(OFCL), the Geophysical
Fluid
Dynamics Laboratory
hurricane model (GFDL),
the
Florida State Superensemble
(FSSE), the five day NHC
statistical
model (SHF5)
and decay
techniques (DSHP), the Met
Office (UKMO), the NCEP
Aviation Model
(AVNO), the Navy
Operational Global
Atmospheric
Prediction System (NGPS),
the statistical climatology
and
persistence model (CLP5),
and no change (NCHG).
(Adapted from Figure 1
of
Davis et al. 2008)
Verification of the inner core structure of the simulated
storms,
examined with a finest mesh of 1.33 km, has proven less
successful. Realistic eye, eyewall and inner spiral
rainband
structures are simulated on the finest mesh (1.33 km; middle
image
below), but
the eye is generally too small and the eyewall
convection too wide. As an example, compare the 4
km
(left) and 1.33 km
(middle) Hurricane Katrina (2005) reflectivity patterns at
0000 UTC 29
August (48h forecast) to the NOAA/AOML/HRD P-3
lower
fuselage
radar image (~1.5 km resolution) at 2317 UTC 28 August
(right).
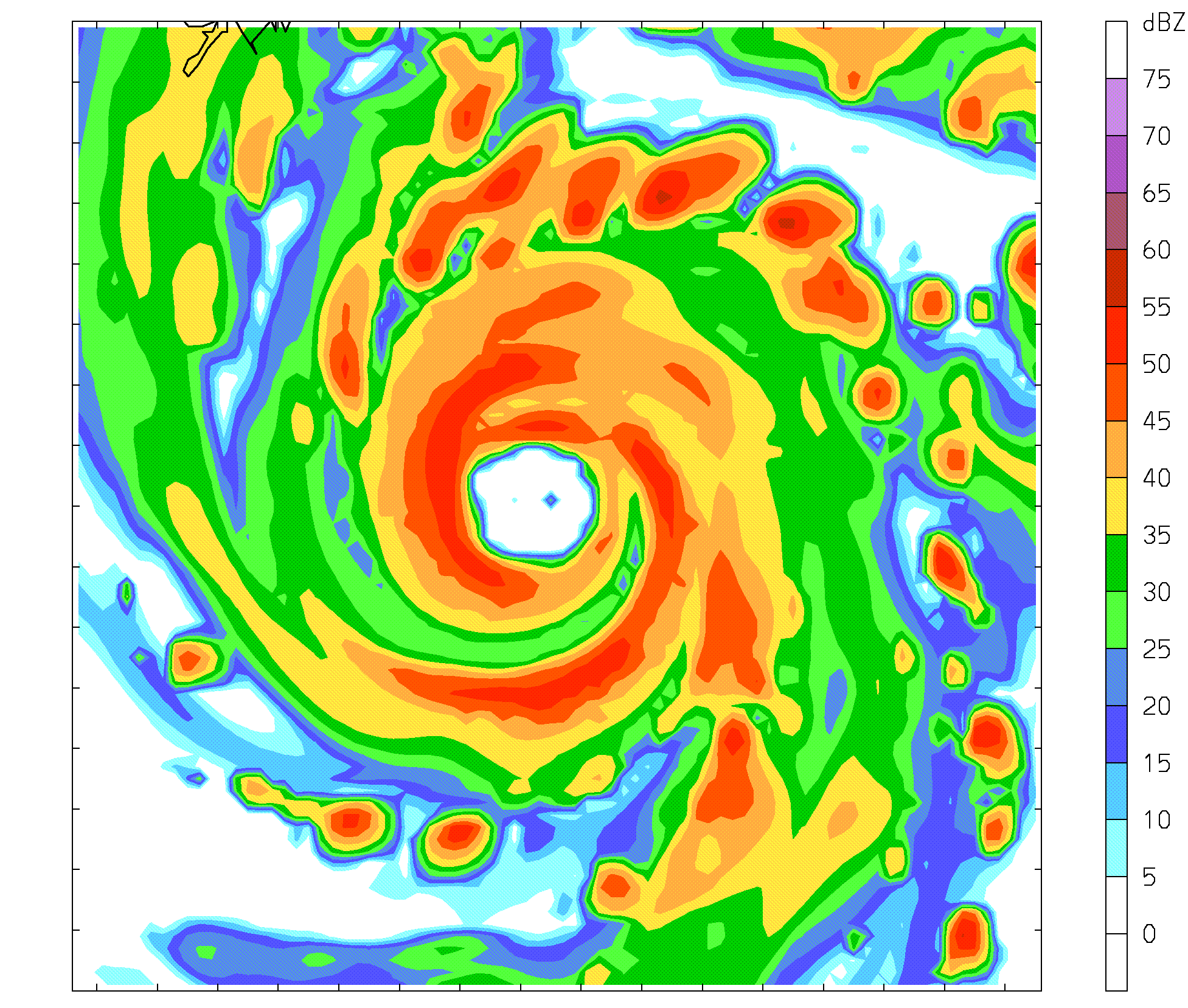
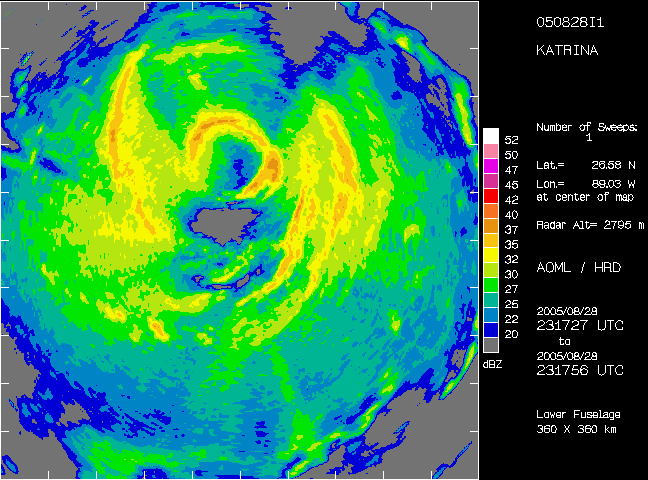
The
scales
of the images are nearly identical with both WRF images
being
320 km on
a side and the P-3 radar being 360 km.
An
unusually
large number of polygonal eyewall shapes were noted
on both the 4 km and 1.33 km grids, as seen in the 1.33 km
Katrina
reflectivity plot above (middle) and 4 km Hurricane Rita
(2005)
reflectivity (dBZ; left) and precipitable water (cm; right)
plots shown
below
at 0300 UTC 24 September (39h forecast).
*** Additional times from both of these runs
are shown in
the "Loops" section below. ***
The
vertices
of the polygonal eyewalls (three in the case of the Rita
triangular eyewall above) show up equally well in the fields
of radar
reflectivity and
precipitable
water (the column integrated water vapor) and thus a
combination of
both are shown in
the animations below highlighting the ubiquitous nature of
elliptical,
triangular, square, and higher wavenumber polygons in the
eyewalls of
WRF simulated TCs across different resolutions and
incarnations of the
model.
Reflectivity
& Precipitable
Water Loops
~
Hurricane Rita,
real time AHW
2.1, 00 UTC 23 September - 12 UTC 24 September (forecast
hours 12-48), 4 km
precipitable water
*
A triangular eyewall is evident throughout most of the
run, becoming
more distinct as Rita approaches the Texas
coast
~
Hurricane Rita,
retrospective AHW
2.1, 18 UTC 21 September - 12 UTC 23 September (forecast
hours 18-60), 1.33
km precipitable
water
*
Triangles, squares and pentagons abound in this higher
spatial
resolution animation
~ Hurricane Wilma,
real time AHW
2.1, 00 UTC 20 October - 00 UTC 24 October (forecast
hours 01-96), 4 km
Reflectivity
*
Note the triangular eyewall from 21 UTC 20 October - 04
UTC 21 October
(frames 21-28) and again after 00 UTC 23 October (frames
72+)
~
Hurricane Katrina,
retrospective AHW
2.1, 00 UTC 28
August - 11 UTC 29 August (forecast hours 24-59), 1.33
km precipitable
water
*
Note the square eyewall from 1700-2000 UTC 28 August
(frames 18-21)
that transitions to a triangle at 2100 UTC
~
Hurricane Katrina,
retrospective AHW
2.2, 00 UTC 28
August - 12 UTC 29 August (forecast hours 24-60), 1.33
km precipitable
water
*
This newer version of the model has fewer squares, more
rounded
quadrilaterals, and a spectacular triangular eyewall on
29 August
(frames 26-29)
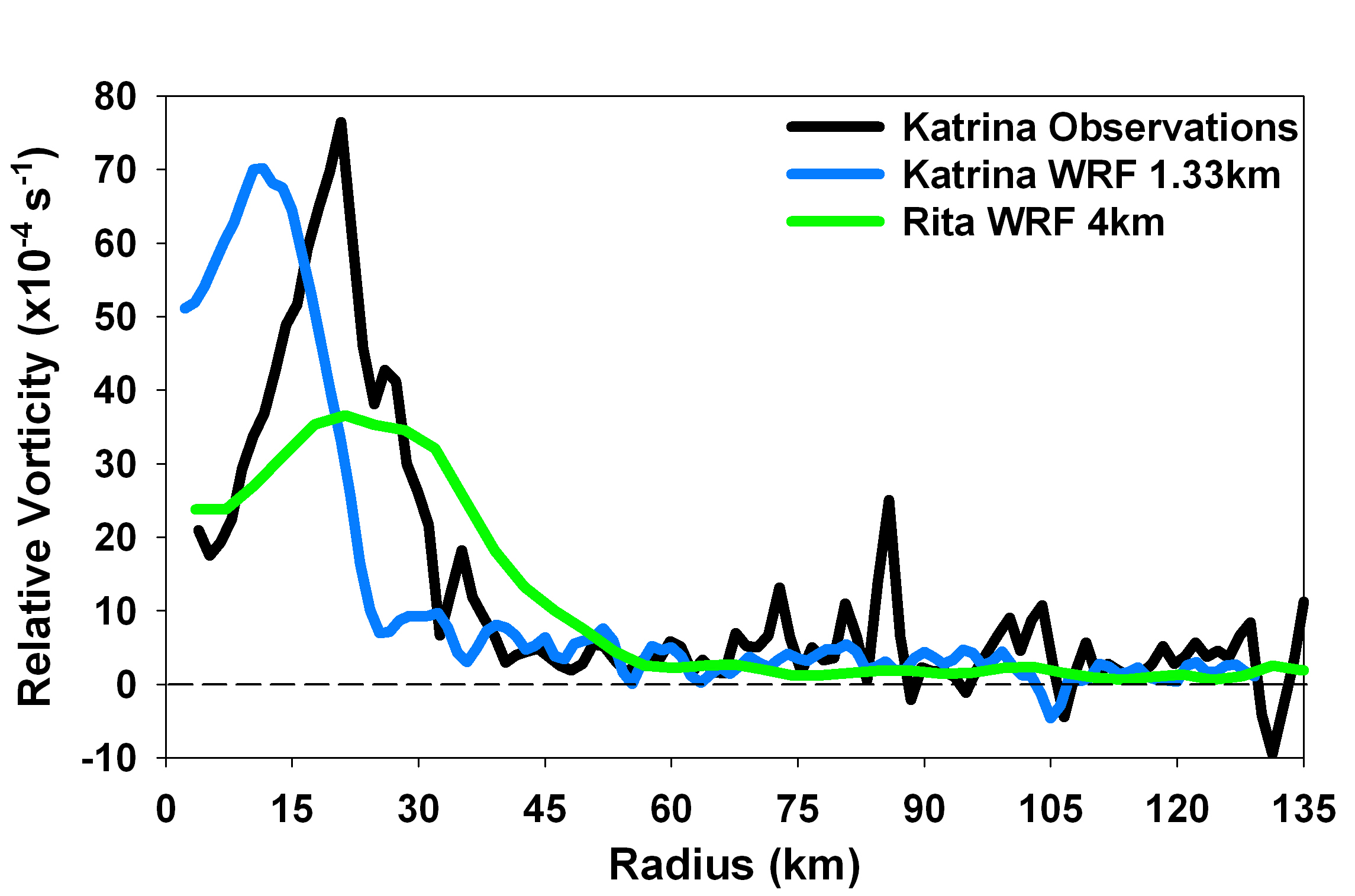
Polygonal
eyewalls have
been documented since the first radar images
of
TCs were
captured (e.g. Lewis and Hawkins 1982), but only
recently has
their
existence been explained using barotropic
instability in a series
of
papers by Wayne Schubert and James
Kossin (Schubert et al.
1999;
Kossin
and Schubert 2001, 2004; Kossin
et al. 2002,
2004; ), although the
idea was first proposed by Muramatsu
(1986).
Briefly, mature and rapidly
intensifying TCs exhibit elevated rings of potential
vorticity (or
relative
vorticity; see image at right) on the inner edge of
the eyewall (Kossin
and
Eastin 2001; Mallen et al. 2005). Analogous to
Rossby waves in
the large
scale circulation of the Earth, counter-propagating
waves with respect to
the flow (deemed
vortex
Rossby
waves
or VRWs; Montgomery and
Kallenbach 1997) exist on the oppositely signed
radial gradients
of
potential vorticity (PV).
If these waves become
phase-locked, they grow
in concert and lead to the exponential
instability of the ring, whereby
the
eyewall vorticity pools into discrete areas, creating
mesovortices (see Figure 3 of Schubert et al.
1999). Depending
on the initial conditions of
the PV
ring, the mesovortices either merge over time and relax to a
monopole
(Schubert et
al. 1999; Chen and Yau 2003), or remain
separate to form
a
quasi-steady,
cyclonically-rotating lattice of vortices that
give the
appearance of
elliptical (two
mesovortices), triangular (three mesovortices)
or
polygonal (four or more mesovortices)
eyewalls
(Kossin and Schubert 2001).
While
most of
the polygonal shaped
eyewalls
are short lived (< 2-3 hr) and change
readily
between
shapes
and intervals of roundness in
both nature
and the WRF model,
TCs (real
and simulated) will
sometimes "lock on" to shapes for
several
hours such
as the
starfish in
Hurricane Isabel (2003) (left; Kossin and
Schubert 2004)
and the triangle or squares
and triangles in the WRF simulated Rita
and Katrina,
respectively. Higher temporal
resolution
model output (every 10 min) was generated for these
instances so the
direction
and rate of rotation of the vertices
could be determined
and
compared to theory and
previous observational studies of
polygonal eyewalls.
High temporal
Resolution (10
min) Precipitable Water Loops
~ Hurricane Katrina,
retrospective AHW 2.1,
12-18 UTC
28 August (forecast hours 36-42, every 10 min), 1.33
km
precipitable water
*
Squares and pentagons abound in this higher time
resolution loop
~
Hurricane Katrina,
retrospective AHW 2.2,
01-03 UTC
29 August (forecast hours 49-51, every 10 min), 1.33
km
precipitable
water
*
A long lived triangular eyewall appears
to rotate clockwise in this newer version of the model
In the loop above, a distinct maximum in eyewall
precipitable
water (PW) can be traced cyclonically around from due
west of the
center at 0140 UTC, to south of the center at 0150
UTC, east at 0200
UTC, north at 0210 UTC and northwest at 0220
UTC. The feature can
also been seen as a local maximum
in eyewall relative
vorticity and thus can be identified as an
eyewall
mesovortex.
The mesovortex completed one full rotation of the
eyewall at a radius
of 20 km in just over 40 min, with a speed of ~50 m s-1.
Assuming
this feature behaves as a linear edge wave (Lamb
1932), since
it's propagating on the very steep vorticity gradient
shown above, it
should have a speed of C = Vmax(1-1/n),
where Vmax
= the maximum tangential wind speed = 70 m s-1
and n =
azimuthal wavenumber = 3, or C = 47 m s-1.
Thus, it
appears this wavenumber 3 feature is indeed an eyewall
mesovortex that
exhibits the propagation characteristics of a PV edge
wave, consistent
with the studies of Kuo et al. (1999) and Reasor et
al. (2000).
It remains to be seen, however, if the real Hurricane
Katrina (2005)
exhibited such persistent eyewall mesovortices.
The very active 2005 Atlantic TC season was
fortunate enough to feature
the
Hurricane Rainband and Intensity Change
Experiment (RAINEX)
which was designed to explore the dynamic
relationships between the
eyewall, inner spiral rainbands
and intensity change in tropical
cyclones. Extensive
observations were collected in both Katrina and Rita
and copious
amounts of data are available to validate our
WRF
simulations against and to investigate whether the
storms actually
featured the realistic looking polygonal
eyewalls simulated with
the
model.
Below
is a
series of reflectivity images from the NOAA
P-3
lower
fuselage
radar in Katrina
at 1753, 1931, 2233, and 2347 UTC 28 August as the
plane was passing through the eye. While the
observations do show
a few bumps and undulations along the inner edge of
the eyewall
reflectivity gradient (especially evident at 1753 UTC;
far left), there
are no indications of any polygonal eyewalls, in stark
contrast to all
of the WRF runs of Katrina above that show distinct
squares or
triangles
throughout the period of study.
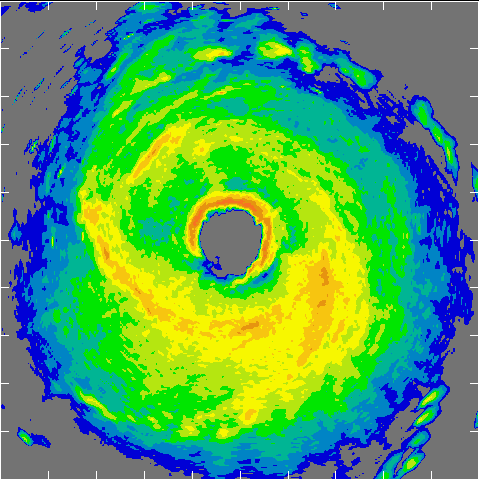
There
are also
no triangles or squares, but perhaps an ellipse at
2153 UTC (far
right), in
the
reflectivity images below of Hurricane
Rita at
1658, 1744
and 2153 UTC 23 September.
Hurricane
Wilma also
shows no indications of a triangular eyewall in
this LOOP of
lower fuselage
radar
images from 1842-2309 UTC 20 October. This
is in stark contrast
to plot to the left which
shows the 23 h forecast of precipitable
water from the real
time, 4 km WRF run of Wilma initialized
at 00 UTC 20 October (the same run shown in
the Wilma
reflectivity loop above).
The first two images of the
loop do, however, show some straight line edges to
the eastern half of
the
eyewall and there is a hint of a pentagon- or
hexagon-shaped eyewall
at 2019 UTC.
AOML/HRD
Doppler
wind
analyses of the inner ~100 km are also
available for Katrina
(below left) and Rita (below right) at .5 km
(bottom two frames of each
panel) and 3 km (top two frames of each panel)
altitudes and can be
compared to the model
predicted wind speeds. Two individual
flights across the storm
center have been overlaid in the figures below to
give more complete
coverage of the inner 100 km to compare to the WRF
output. The
color scale of the wind speed has been matched as
closely as possible
between the HRD generated plots (right side of
each panel) and the WRF
output plotted with GEMPAK (left
side of each panel).
Left:
Katrina HRD composite winds 1725-1946 UTC and AHW
2.1 1.33 km winds at
1800 UTC 28 August.
Right: Rita HRD composite winds 2015-2208 UTC 23
September and AHW 2.1
4 km winds at 01 UTC 24 September.
The geometric patterns and distinct
maxima
in reflectivity, precipitable water and relative
vorticity discussed
above also stand out clearly in the total wind
field, especially at .5
km (bottom right frame of each panel). No such
wavenumber 3
(triangle; Rita, right) or 4 (square; Katrina, left)
asymmetries show
up in the observations, which reveal more of a
wavenumber 1 pattern
with maximum winds north and northeast (downshear to
downshear right)
of the center. This pattern is captured by the
AHW simulations at
the 3 km level (top right frame of each panel), as
is the larger radius
of hurricane strength winds in the northwest
quadrant. The radial
profile of the winds, however, is much too narrow
(i.e., the wind speed
decreases much too quickly with radius) in all
quadrants except to the
southwest.
Finally, the AHW 2.1 1.33 km simulated eye size is
consistently too
small (see above left plots for Katrina), while the
4 km simulated eye
size (see above right plots for Rita) is much closer
to the
observations recorded in both storms.
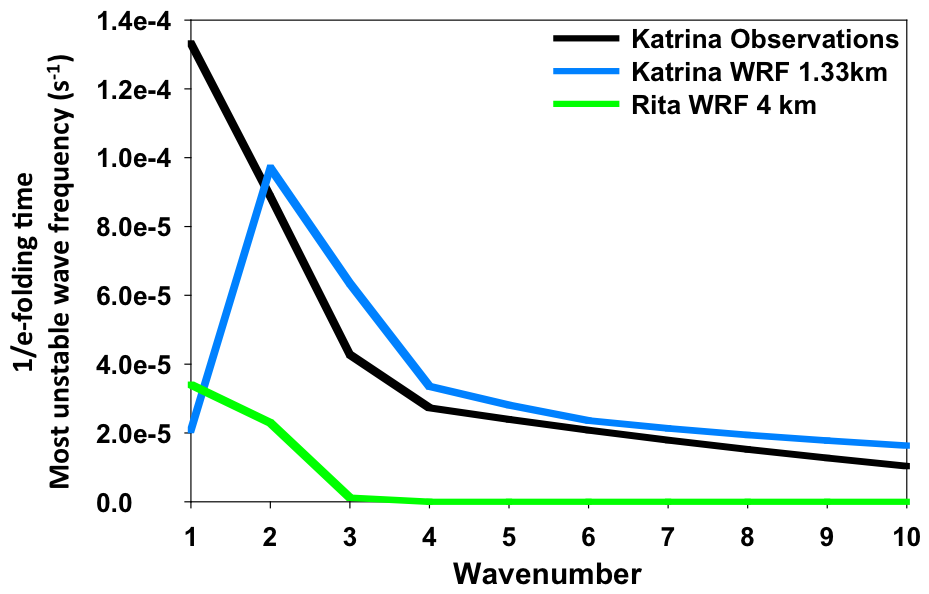
Because of the difference between the simulated and
observed radial
profiles of tangential wind speed noted above and
shown in the left
panel above, it is possible that the AHW simulated
Katrina and Rita may
support higher wavenumber asymmetries than the real
storms. To
address this question, a linear stability analysis
(code provided by M.
Bell and M. Montgomery of the Naval Postgraduate
School) of the radial
profiles of relative vorticity (see radial vorticity
plot next to the
discussion on VRWs and mesovortices above) was
conducted.
As can be seen in the right panel above, the 1.33 km
WRF simulation of
Katrina is most unstable to wavenumber 2, with
significant wavenumber
1-4 instability, while the observations show the
real Katrina was most
unstable to wavenumbers 1 and 2. The 4 km WRF
simulation of Rita
is most unstable to wavenumbers 1 and 2, with very
little instability
at higher wavenumbers, consistent with its wider
vorticity peak and
gentler vorticity gradients (Schubert et al.
1999). Thus, the
linear stability analysis reveals no "smoking guns"
when it comes to
the fastest growing modes being the wavenumber
asymmetries most often
seen in the simulations. Other possible
explanations for the
exceeding active wavenumber 3 and 4 asymmetries in
the simulated storms
may be insufficient cloud microphysical schemes,
model resolution or
Cartesian grid geometry. These options are
currently being
explored in the AHW model.