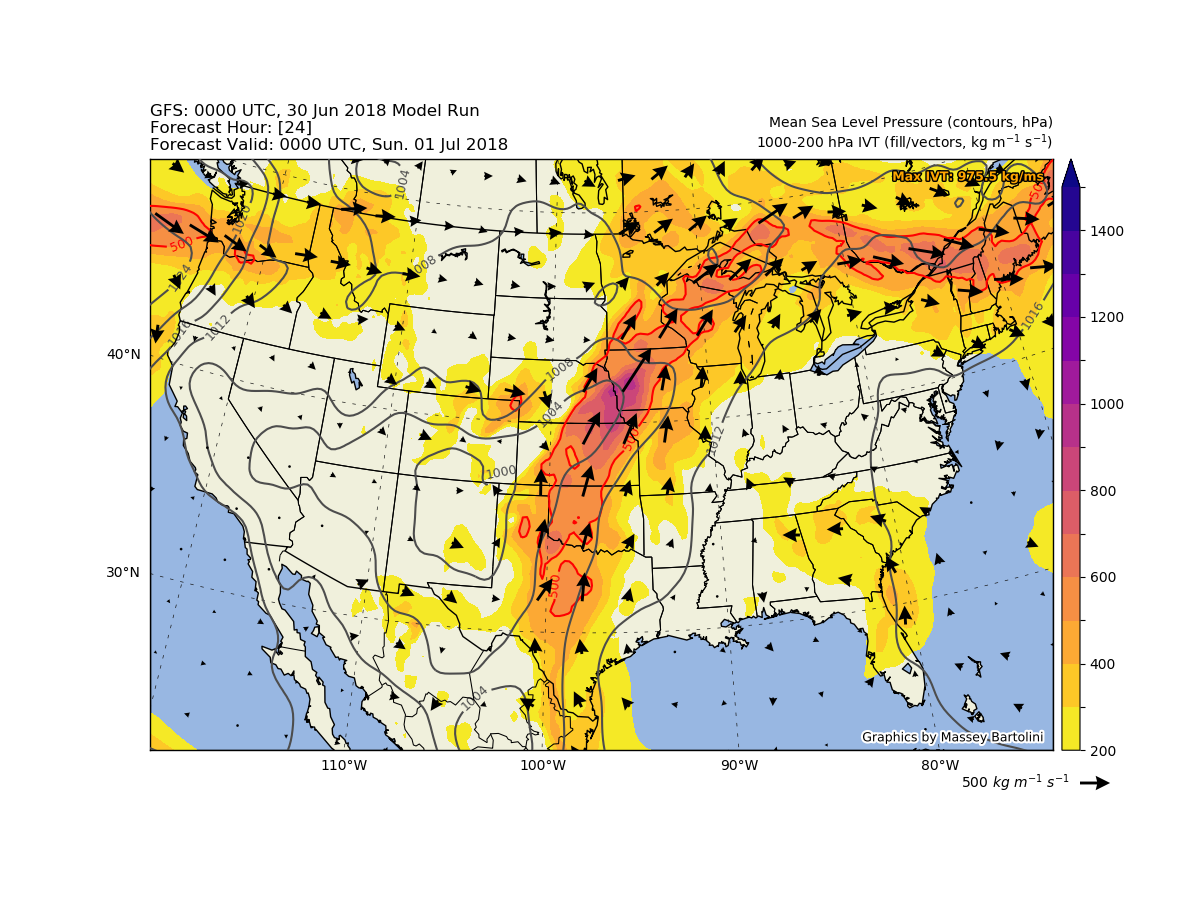
Synoptic-scale GFS plots of dynamic and moisture-related variables.
Winter weather presents numerous forecast challenges for convection-allowing models (CAMs), particularly in complex examples such as lake-effect snow and orographic precipitation, due to winter weather's mesoscale variability and uncertainties in model physics parameterizations. Current CAM ensembles used in NWS operations, such as the HREF, are constructed from individual CAM forecasts. However, accurate and reliable probabilistic forecasts are challenging to make from these ensembles if not all members are equally likely, due to physics biases and other sources of model error.
My M.S. project was part of the Collaborative Science, Technology, and Applied Research (CSTAR) program between the National Weather Service (NWS) and the University at Albany. In addition to the support of the PIs for my project here at UAlbany, I also collaborated with forecasters at the NWS Forecast Offices in Albany, NY, and Buffalo, NY, and model developers at the NOAA Earth System Research Laboratory in Boulder, CO, to gain insights on lake-effect forecasting challenges from their perspectives.
Thesis Title: Convection-Permitting Ensemble Forecasts of the 10–12 December 2013 Lake-Effect Snow Event: Sensitivity to Microphysical, Planetary Boundary Layer, and Surface Layer Parameterizations.
Thesis Abstract:
Lake-effect snow (LeS) presents a substantial forecast challenge for convection-permitting models, due in part to uncertainties in the parameterization of microphysical (MP) and planetary boundary layer / surface layer (PBL/SL) processes. Here we focus on understanding these uncertainties for a LeS event that occurred during 10–12 December 2013 during the Ontario Winter Lake-effect Systems (OWLeS) field campaign. Throughout this event, long-lake-axis-parallel snowbands persisted downwind of the eastern shore of Lake Ontario, leading to snowfall accumulations as high as 105 cm (liquid precipitation equivalent of 64.5 mm) on the Tug Hill Plateau.
We run nested simulations of the 10–12 December 2013 LeS event at 12-, 4-, and 1.33-km horizontal grid spacing using the Weather Research and Forecasting (WRF) model configured similarly to the operational High-Resolution Rapid Refresh (HRRR) model. Two suites of sensitivity experiments are conducted for the event, first, with nine different MP schemes, and second, with eleven different PBL/SL schemes. Large differences between the MP experiments are found in the LeS band intensity and precipitation type, with smaller differences in the band timing, position, and morphology. Maximum storm-total liquid precipitation equivalent amounts among MP ensemble members range from 35 to 62 mm. Results from the WRF simulations are compared to detailed observations from OWLeS, including scanning and profiling radar data and surface snowfall and crystal habit observations. Additionally, measurements from the University of Wyoming King Air aircraft, including vertically pointing cloud radars and in-situ flight-level thermodynamic and microphysical observations, are used to compare modeled and observed cloud structures. By analyzing cloud microphysics, such as supercooled liquid water content, hydrometeor size distributions, and precipitation fallspeed, we compare modeled and observed lake-effect cloud properties and assess which MP schemes are most accurately simulating cloud processes during this LeS event.
Motivated by substantial differences in the model-observational comparisons, we also perform several sensitivity experiments with the aerosol-aware Thompson MP scheme used in the HRRR, modifying the snow size distribution and fallspeed within WRF to quantify single-scheme precipitation uncertainty. These sensitivity experiments vary maximum liquid precipitation equivalent amounts over the Tug Hill Plateau by 5 to 15 mm relative to the Thompson control simulation.
In contrast to the MP ensemble experiments, the PBL/SL ensemble primarily shows differences in the LeS band intensity and morphology. Maximum storm-total liquid precipitation equivalent amounts among PBL/SL ensemble members range from 54 to 99 mm, which is a larger range than in the MP ensemble. PBL/SL precipitation forecasts are compared to observations of manual and radar-estimated precipitation to identify which PBL/SL schemes produce excessive QPF. Several sensitivity experiments are also performed with the Quasi-Normal-Scale-Elimination (QNSE) SL scheme, which produces the highest fluxes and snowfall, by varying the Prandtl number and critical threshold for friction velocity to investigate surface flux sensitivity.
As a participant in the 2015 National Weather Center Research Experiences for Undergraduates program, I completed a summer research project on Sierra Nevada winter storms under the mentorship of NSSL/CIMMS scientist Dr. Heather Reeves.
Abstract: Knowing the liquid-water fraction (LWF) in the rain/snow transition zone has useful applications in hydrology, road
transportation, and aviation. Several control parameters that dictate the LWF are identified through a series of idealized, two-dimensional
experiments using a spectral-bin microphysical model. These are the stability, the drop-size distribution (DSD), and the degree of riming (RF).
The most important of these is stability. Though DSD and RF have secondary impacts, they are still non-negligible. In real case studies, stability
can be determined from numerical-model output, but DSD and RF are unknown. Therefore, several sets of experiments are conducted to gauge the effects
of DSD and RF variations on the rain/snow transition zone for actual case studies of orographic precipitation in the western United States. Each
experiment uses a unique combination of DSD and RF, which are assumed to be constant across the entire domain. These experiments show that DSD and
RF have nontrivial effects on the LWF and depth of the rain/snow transition zone. In some case studies, the depth of the transition zone varies by
as much as 450 m.
I presented this research as a poster in January 2016 at the AMS Annual Meeting in New Orleans, LA, and as a presentation in April 2016 at the National Conferences for Undergraduate Research in Asheville, NC.