Verification
of
Daily Thunderstorm Occurrence Using the
National Lightning Detection
Network (NLDN)
Since 1983, a
daily thunderstorm
probability
forecast contest has been organized by the
Department
of
Atmospheric and Environmental Sciences at the
University at Albany
during the summer months of June, July, and August
(Bosart and Landin 1994).
The contest involves predicting the probability to the
nearest 10% that
a thunderstorm (TS) will be reported during a 24 hour
period (starting
@ 18
UTC) at ten locations across the continental United States
(see table
below). The
forecasts are verified by standard METAR reports and are
scored against
consensus and a 16 year thunderstorm climatology
(1988–2003) shown in
the table below as the percentage of days per month with a
METAR TS
report.
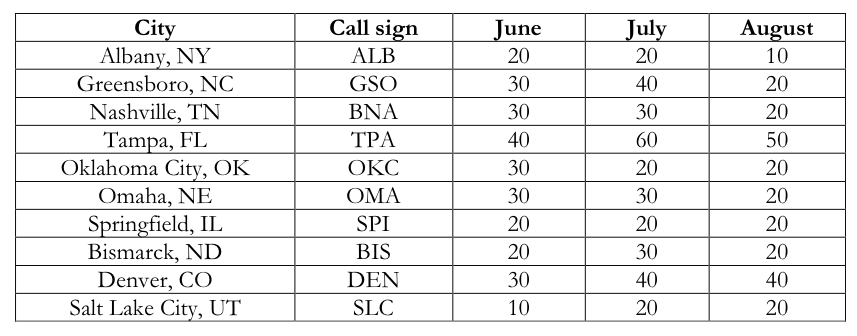
In recent years however, there have been several
instances,
particularly during the overnight hours, in which a
thunderstorm failed
to
be reported in the METAR reports despite its occurrence
(see 2009
WAF
Conference presentation for examples). During
such instances, the forecast contest was verified by
contacting the
attendant NWS office directly and/or examining WSR-88D and
National
Lightning
Detection Network (NLDN) data. Given its
continuous
space and time coverage, an average detection efficiency
of ~95% and
mean location errors of < 500 m (Cummins and Murphy
2009), using the
NLDN to verify thunderstorm occurrence is explored at the
original 10 stations of the Albany forecast contest, plus
10 additional
cities to cover the full spectrum of summertime, synoptic
environments
and rates of thunderstorm occurrence across the
continental United
States.
Locations
(by
METAR station ID) of the 20 cities examined, overlaid on
the 15 year
(1995-2009) June, July, August NLDN flash density (in
flashes per km2
per year).
The
tables and bar
charts below show the 15 year (1995-2009) June, July
and August (JJA)
METAR
thunderstorm climatologies (ASOS column) and the
climatology of NLDN
detected lightning within 5, 10, 15 and 20 km of
each station as the
fraction of days per month with a TS report or NLDN
detected
flash. The red numbers highlight the
radial bin with the
NLDN climatological value closest to the ASOS value
(i.e., for June
ALB, .16 at 10 km is the closest to the .15 ASOS
value). Numbers
in blue highlight instances where the 5 km NLDN
climatological value
exceeds the ASOS value.
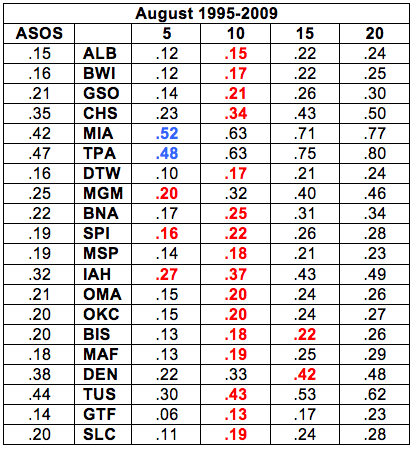
As seen in the tables and figures
above, 10 km is
the radial
range that best agrees with the
METAR TS
climatology, in
agreement with Bosart and
Landin's (1994) statement
that
thunder can generally be heard
within 10 km of a
station.
There are, however, some distinct
exceptions: 1) MIA
and
TPA both have months (July and
August) where the
number
of days with flashes within 5 km
of the station
exceeds the
number of TS days, and 2) TS
reports for stations in
the
western U.S.
(DEN, BIS, SLC) tend to agree best with the
number of NLDN lightning days
further from the
stations,
e.g., at the 15
km radius.
The tables and graphs above
explore the number of METAR
TS and NLDN detected lightning days without taking
into account whether
these days actually match in the two data
sets. The
tables below take date matching into account and
address two
fundamental questions: 1) If the NLDN detects
lightning within X km
(where X = 5, 10, 15 or 20) of a station, what
percentage of the time
does that station report a TS on that day?,
and 2) If a TS is
reported by a station, what percentage of the time
does the NLDN detect
lightning within X km of the station on that
date? Average values
are listed at the bottom of the tables and red
(blue) numbers denote
values one standard deviation above (below) the
mean.
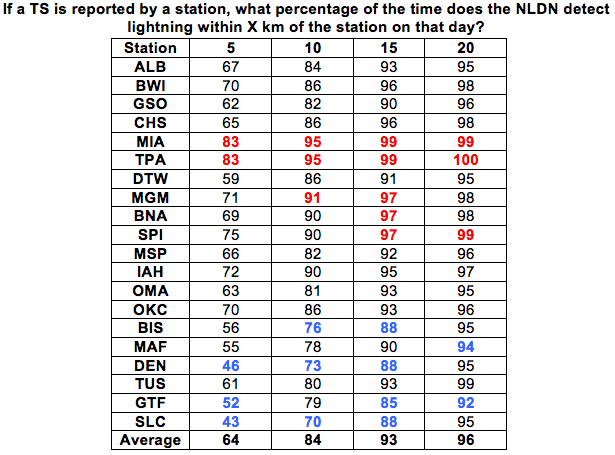
The table on the left above shows
that GSO, CHS, MSP,
OKC,
and especially OMA and DEN are excellent verifiers
of thunderstorms,
e.g., when there is lightning within 10 km of the
station, a TS is
reported in the METARs. On the other hand,
MIA, TPA, TUS and GTF
are very poor verifiers of thunderstorms, i.e. there
are many days with
lightning within 10 km of the station when no TS is
reported.
While one might suspect that the low verification
rates at TPA and MIA
are due to the fact that lightning is observed
nearly every summer day
in
Florida, the flash density plot above shows that
stations such as IAH
and CHS have almost as lightning per year as MIA and
TPA and verify at
rates above the mean. (Possible explanations
for the low
verification rate in TUS and GTF will be explored
below.)
The table to the right above verifies the strong
east/west dichotomy
noted
above: stations in the western U.S. (DEN, SLC, BIS,
GTF) verify TSs
with lightning at much farther distances from the
stations than
locations
in the eastern U.S.. Fewer trees and buildings
for longer sight
lines and less sound absorption are the most likely
contributing
factors to this difference.
The
next factor
explored is the interannual variability of the total
number of JJA METAR reported TS days (hereon denoted
METAR) and total
number of JJA days with lightning detected with 10
km of each
station (NLDN10),
and their
correlation.
The table to the right shows the Pearson (r) and
Spearman rank (rho)
correlation coefficients between METAR and NLDN10;
the number of years,
out of 15, NLDN10 > METAR (mean of 7.3); and the
average absolute
value of NLDN10-METAR, i.e., the number of days
difference between the
two measures of thunderstorm activity (mean = 4.9
days, median = 3.3
days).
In the correlation columns, the light blue, medium
blue, dark blue and
purple
shadings represent cities failing to have a
significant positive
correlation between METAR and NLDN10 at the 99.5,
99, 97.5 and 95%
levels, respectively. In the last two columns,
red (blue) numbers
represent
values one standard deviation above (below) the mean
values indicated
in the paragraph above.
A number of interesting results are revealed in the
table. First,
MIA, TUS, BNA, MGM, GTF and ALB all fail to have
significant positive
correlations between the number of JJA METAR
reported TS days and the
number of days with lightning within 10 km of the
stations. On
the other end of the spectrum, OKC, DEN, BWI, and
IAH have very high
correlations between the two data sets.
Second, the fourth column
is another confirmation of western stations having
METAR TS days with
lightning at much greater distances than eastern
stations. Third,
the last column shows that the largest average
differences between
METAR and NLDN10 are recorded at MIA and TPA, where
lightning routinely
is detected within 10 km of the stations and no TS
is reported, and DEN
and TUS, where TSs are verified with lightning at
distances > 10 km
and monsoonal variability dominates,
respectively. Stations with
low differences are DTW, MSP and OKC, indicating 10
km is the ideal
radial distance for TS verification at those sites.
The plots below examine the points above in greater
detail by showing
the interannual variability in METAR (solid lines)
and NLDN10 (dashed
lines) for six selected cities (plots for the other
14 cities can be
found here).
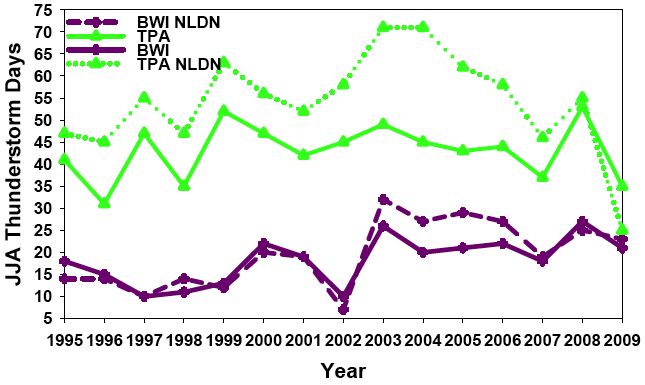
The plot to the left above shows
the interannual
variability of METAR and NLDN10 for the cities with
the two lowest
correlation coefficients between the data sets, MIA
and TUS. The
poor correlation in TUS seems to be due to changes
in METAR lagging
those in NLDN10 by a year. The strong
interannual variability of
precipitation in Arizona associated with the North
American Monsoon
System (NAMS) seems to be the major contributing
factor in this
difference and is explored further below.
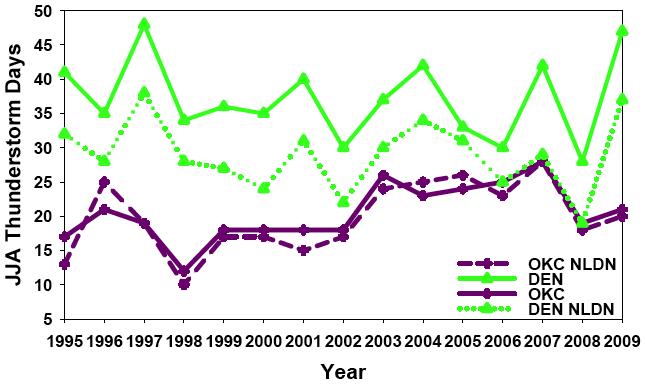
The low correlation in
MIA is due to METAR
remaining flat
with time, while NLDN10 (which is
always larger than
METAR) exhibits significant year
to year
variability. In
contrast, TPA (shown to the right
above), which also
always
has NLDN10 > METAR, does
exhibit variability in
both
METAR and NLDN10 and has a
significant positive
correlation between the two.
BWI
(above right), DEN
and OKC (both left) exhibit three
of the largest correlation
coefficients between
METAR and
NLDN10 despite large differences
in number of TS
days at
each station and between METAR
and NLDN10 at DEN
where METAR is always greater
than NLDN10.
The next subject explored is the relationship
between summertime
thunderstorm activity over the U.S. and ENSO.
The table below
shows the Spearman rank correlation coefficients
between the two
measures of TS activity (METAR and NLDN10) and the
preceding winter
(DJF) and spring (MAM), and concurrent (JJA) Oceanic
Niño
Index (ONI). The colored numbers
indicate
statistical significance at the values listed below
the table.
The city with the strongest and most consistent
relationship with ENSO
is TUS, exhibiting negative correlations (i.e., warm
Pacific waters,
less TS activity) across the seasons and measures of
TS activity, with
4/6 values being statistically significant. DTW,
BNA and MSP
show positive correlations between winter and spring
ENSO values and
subsequent JJA TS activity, while GSO,
MGM, MAF and
DEN all exhibit statistically significant positive
correlations between
TS activity and ENSO in JJA. These later
relationships, and the
one with TUS noted above, are broadly consistent
with the known
patterns of JJA ENSO precipitation anomalies shown
below with less
rainfall/TS activity in the midwest, upper Plains
and southwest, and
more rainfall/TS activity in the southeast and Great
Plains (the top
panel is from this study with pink/red = positive
correlations and
blue/navy = negative correlations; and the bottom
panel is from CPC).
Finally,
relationships involving the amount of TS activity
between the 20 cities
are examined. The two tables below show the
Spearman rank
coefficients for the correlations of NLDN10 (top)
and METAR (bottom)
between each city with the significant values
colored as indicated
within the tables.
The cities with the largest numbers of significant
correlations are OKC
and IAH, which reveal more TS activity in these
cities is positively
correlated with activity in cities on the east coast
and in the
southeast and negatively correlated with TS activity
in the upper
midwest, northern Plains and western U.S. (MGM and
MAF have fewer
significant correlations but also reveal the same
pattern).
Conversely, SPI TS activity is positively correlated
with activity in
the upper midwest and northeast, and strongly
negatively correlated
with activity in the southeastern U.S. and southern
Plains.